In this post
Many chemical reactions are irreversible. The reactants once converted into the products, cannot be changed back again. These reactions are represented using an à sign in the equation to show that the reactants can be converted into the products, but the products cannot be converted back into the reactants.
In some chemical reactions the reactants can be converted into the products and, if the conditions are altered, the products can be changed back into the reactants. These are known as reversible reactions. These reactions are represented using the symbol in the chemical equation to show that the reaction can go forwards to form the products or backwards to form the reactants.
Two examples of reversible reactions are the effect of heat on ammonium chloride and the dehydration of hydrated copper(II) sulphate.
Effect of heat on ammonium chloride
Ammonia gas (NH3(g)) and hydrogen chloride gas (HCl(g)) react to produce white crystals of the ionic compound ammonium chloride (NH4Cl(s)). This is an example of a reversible reaction, as when the ammonium chloride salt crystals are heated, they break down to produce ammonia gas and hydrogen chloride gas.
If this reaction is done in a sealed tube, the white crystals of ammonium chloride will disappear from the bottom of the tube to produce two colourless gases and then reappear at the top of the tube. This is because the ammonia and hydrogen chloride gases travel to the top of the tube where they cool. The two gases recombine to form the ammonium chloride again.
The equation for this reversible reaction is shown below:
![Rendered by QuickLaTeX.com \[NH_4Cl_{(s)} \Longleftrightarrow NH_{3(g)} + HCl_{(g)} \]](https://b3801007.smushcdn.com/3801007/wp-content/ql-cache/quicklatex.com-2353738654c0d5471cf8f4458c68af45_l3.png?lossy=2&strip=1&webp=1)
Dehydration of hydrated copper(II) sulphate
Hydrated copper(II) sulphate crystals are blue. The formula of hydrated copper(II) sulphate is CuSO4.xH2O. The dot used before the xH2O in the formula shows that the water molecules are not bonded to the copper(II) sulphate. These water molecules are known as water of crystallisation and can be easily removed through heating the copper(II) sulphate crystals until the water evaporates away.
Once all of the water of crystallisation has been removed, the product is anhydrous copper(II) sulphate which is white. The water can be collected using the equipment set-up in the diagram below:
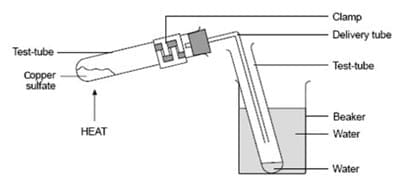
When the hydrated blue copper(II) sulphate has turned white, all of the water has been removed. The water has been collected and can be added to the anhydrous copper(II) sulphate. By adding water to the anhydrous copper(II) sulphate we hydrate the white anhydrous copper(II) sulphate crystals and turn them back into blue hydrated copper(II) sulphate. The equation showing that this is a reversible reaction is shown below:
![Rendered by QuickLaTeX.com \[CuSO_{4.}xH_2O_{(s)} \Longleftrightarrow CuSo_{4(s)} + H_2O_{(l)} \]](https://b3801007.smushcdn.com/3801007/wp-content/ql-cache/quicklatex.com-e2dad1d7a624bc2b364cd9a11160bb70_l3.png?lossy=2&strip=1&webp=1)
Dynamic equilibrium
A reversible reaction is one which can move forwards towards the products or in the reverse direction back towards the reactants. For example, reactants A and B react to produce products C and D. Products C and D can also break down to form the reactants A and B as shown by the equation:
![Rendered by QuickLaTeX.com \[A + B \Longleftrightarrow C + D \]](https://b3801007.smushcdn.com/3801007/wp-content/ql-cache/quicklatex.com-38e577df3261f461155fe014ab8aaa1d_l3.png?lossy=2&strip=1&webp=1)
The reaction between A and B occurs on the left side of the equilibrium. The reaction between A and B to produce C and D is known as the forwards reaction. The reaction between C and D occurs on the right side of the equilibrium. The reaction between C and D to produce A and B is known as the reverse reaction.
If a reversible reaction takes place in a sealed container, no products can escape, and no other reactants can get in. This is known as a closed system. An equilibrium exists where there is a state of physical balance. In chemistry terms this means that there is a balance between the forwards and reverse reactions.
A reversible reaction occurring in a closed system can reach a state of dynamic equilibrium.
A dynamic equilibrium means that both forwards and reverse reactions are happening, but they are in a state of balance. The characteristics of a chemical reaction at dynamic equilibrium are that the:
- Forwards and reverse reactions are occurring at the same rate
- Concentrations of the reactants and products remain constant
We can describe the position of equilibrium depending upon the proportions of reactants and products present in the reaction mixture in the closed system. If the equilibrium mixture contains a higher proportion of reactants than products, we can say that the position of equilibrium lies on the left.
If the equilibrium mixture contains a higher proportion of products than reactants, we can say that the position of equilibrium lies on the right.
A reaction will remain in a state of dynamic equilibrium unless factors such as temperature or pressure inside the closed system are changed. If the temperature or pressure is altered, the equilibrium will shift to oppose that change and maintain a state of balance. This shifting alters the position of the equilibrium. It may shift in the forwards or backwards direction to correct the imbalance.
Effect of temperature changes on equilibria
The direction in which an equilibrium will shift depends upon the type of temperature change occurring and the type of reaction. In a reversible reaction if the forwards reaction is endothermic, the reverse reaction is exothermic and vice versa. In the Haber process, nitrogen gas (N2(g)) and hydrogen gas (H2(g)) react to form ammonia gas (NH3(g)). This is a reversible reaction and the ammonia can also break down again to form nitrogen gas and hydrogen gas, as shown by the equation:
![Rendered by QuickLaTeX.com \[N_{2(g)} + 3H_{2(g)} \Longleftrightarrow 2NH_{3(g)} \]](https://b3801007.smushcdn.com/3801007/wp-content/ql-cache/quicklatex.com-0a9aadebd23968c104bf279b460224d8_l3.png?lossy=2&strip=1&webp=1)
The forwards reaction is exothermic which means that the reverse reaction must be endothermic. If the temperature of the closed system was increased, the equilibrium would shift to decrease the temperature again to achieve a balance. In the case of the Haber process, an increase in temperature would force the equilibrium to shift in the endothermic direction to reduce the temperature again. This means that the equilibrium would shift in the reverse direction and the yield of ammonia produced would decrease. If the temperature for this reaction was decreased, the equilibrium would shift in the exothermic direction to increase the temperature and the yield of ammonia would increase.
If the forwards reaction was endothermic, an increase in temperature would cause the equilibrium to shift in the forwards direction to reduce the temperature. A decrease in temperature would cause the equilibrium to shift in the reverse and exothermic direction to increase the temperature again.
Effect of pressure on equilibrium
When gas particles hit the walls of their containers, pressure is created. If there are less particles of gas in the same volume at the same temperature, the pressure will be lower. When the pressure in a closed system is increased, the equilibrium shifts to decrease the pressure. It does this by shifting in the direction that produces the fewest moles of gas.
If the pressure in the closed system was decreased, the equilibrium shifts to increase the pressure by shifting in the direction that produces the most moles of gas. For example, in the Haber process equation there are more moles of gas on the left than on the right:
![Rendered by QuickLaTeX.com \[N_{2(g)} + 3H_{2(g)} \Longleftrightarrow 2NH_{3(g)} \]](https://b3801007.smushcdn.com/3801007/wp-content/ql-cache/quicklatex.com-0a9aadebd23968c104bf279b460224d8_l3.png?lossy=2&strip=1&webp=1)
If the pressure is increased, the equilibrium would shift to decrease the pressure by shifting in the forwards direction towards the right side with the fewest moles.
If the pressure was decreased, the equilibrium would shift to increase the pressure by shifting in the reverse direction towards the left side with the most moles. If the number of moles of gas on both sides of the equation is the same, any change in pressure will have no effect on position of the equilibrium.
Effect of the use of a catalyst on equilibrium
Catalysts speed up the rate of a reaction and work by providing an alternative pathway with a lower activation energy. If a catalyst is used in a reversible reaction, the rates of both the forwards and backwards reactions will be increased equally.
Although equilibrium will be reached much faster, the concentrations of the reactants and products remain equal, so the position of equilibrium does not change.
The effects of temperature, pressure and catalysts are important considerations for chemists in industry when they are deciding on the best conditions to use to achieve the highest yield possible in the shortest amount of time.
Often the conditions used have to be compromise conditions where there is a balance between achieving the best yield possible and having a good rate of reaction. The conditions for the Haber process are:
- A high temperature of 450℃
- A high pressure of 200 atmospheres (200 x normal pressure)
- An iron catalyst is needed
When making ammonia, a high pressure is favoured as it causes the equilibrium to shift in the forwards direction, maximising yield. A higher pressure also increases the reaction rate. However, high pressures also cause issues. Use of pressures higher than 200 atmospheres would produce a high yield of ammonia but would also be very costly. The piping and machinery needed to maintain the high pressures are expensive and would need replacing very often.
The forward reaction is exothermic, thus increasing the temperature will cause the equilibrium to shift in the reverse direction and reduce the yield of ammonia produced. A lower temperature shifts the equilibrium in the forwards direction and increases the yield of ammonia. However, lower temperatures mean a lower rate of reaction. A compromise temperature of 450oC is therefore used to strike a balance between yield and rate of reaction.
An iron catalyst is used to increase the rate of reaction. The catalyst does not change the position of the equilibrium and does not change the yield of ammonia produced.