In this post
When non-metals react with metals, a transfer of electrons takes place and an ionic bond is formed. When non-metals react with other non-metals, neither of them will lose their electrons and both want to gain electrons to achieve the stability of a full outer shell. Ionic bonding is not possible between two non-metal atoms so they must instead form compounds through sharing electrons in a different type of bonding known as covalent bonding.
There is a force of attraction between the positively charged nucleus of the atom and the negatively charged electrons in the outer shell of other atoms. This force of attraction causes the atoms to move closer towards each other until their electron shells are overlapping.
This overlapping of electron shells means that the electrons in the outer shell can be shared by both atoms, and both atoms therefore have a full outer shell of electrons as long as the atoms stay bonded in this way. There is an electrostatic force of attraction between the nuclei of the two atoms and the electrons being shared between them. This force is known as a covalent bond.
A covalent bond is formed when atoms share a pair of electrons. Any compound formed as a result of non-metal atoms forming covalent bonds, is known as a covalent compound.
Dot-and-cross diagrams
Dot-and-cross diagrams can be drawn to represent covalent bonds in a range of covalent compounds. In the dot-and-cross diagrams for covalent compounds, only the outer electrons are shown. You may see some that include the inner electrons but these are not necessary as it is only the outer electrons which are involved in the formation of the covalent bonds.
Some dot-and-cross diagrams may also not include the circles of the electron shells and show only the electrons in the outer shell.
You should be able to use dot-and-cross diagrams to represent covalent bonds in:
- Diatomic molecules (including H2, O2, N2, halogens and hydrogen halides)
- Inorganic molecules (including water, ammonia and carbon dioxide)
- Organic molecules containing up to two carbon atoms (including methane, ethane, ethene and those containing halogen atoms)
There is a very quick way to find out the number of convalent bonds that an element will form – we can take the group number of the element from 8. For example, carbon is in group 4 in the periodic table so it will form 4 () bonds.
Diatomic molecules
A diatomic molecule is one which is made up of only two atoms covalently bonded together. These can include hydrogen, oxygen or other examples.
- Hydrogen gas (H2) – each hydrogen atom has one electron in its outer shell and needs to gain one more to achieve stability. One hydrogen atom will share its outer electron with another hydrogen atom to form the diatomic molecule H2. A single covalent bond is formed between the two hydrogen atoms where one pair of electrons is shared. The dot-and-cross diagram for H2 is shown below
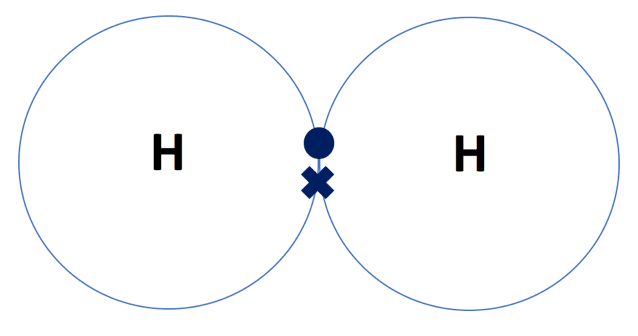
- Oxygen gas (O2) – each oxygen atom has six electrons in its outer shell and needs to gain two to achieve stability. Each oxygen atom in the pair will share two of their electrons, meaning that two pairs of electrons are shared between them forming a double covalent bond, as shown in the dot-and-cross diagram below
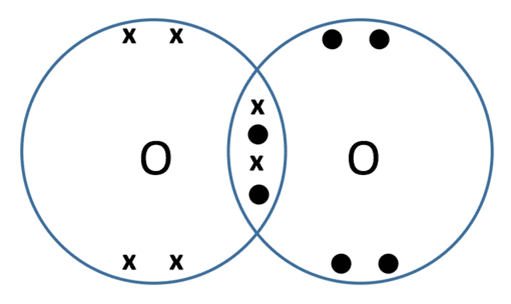
- Other examples of diatomic molecules include nitrogen gas (N2), halogens such as chlorine (Cl2) and bromine (Br2) and hydrogen halides such as hydrogen chloride (HCl) and hydrogen iodide (HI)
Inorganic molecules
- Water (H2O) – oxygen atoms have six electrons in their outer shell. While they sometimes form ionic bonds, they can also form covalent bonds by sharing two electrons with other non-metal atoms. In water molecules, the oxygen shares electrons with the two hydrogen atoms. The dot-and-cross diagram for water is shown below
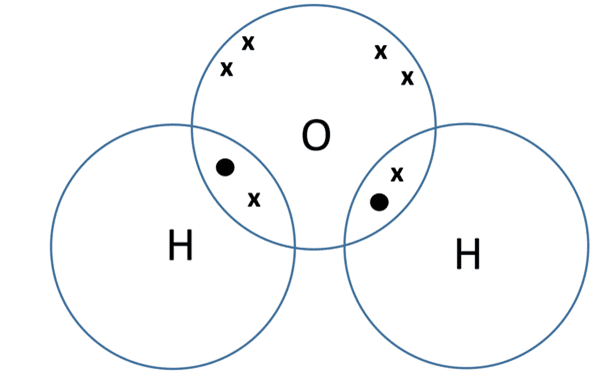
- Ammonia (NH3) – nitrogen has five electrons in its outer shell so we know it needs to form three covalent bonds to make up the extra electrons needed. The three covalent bonds are formed to hydrogen atoms in ammonia, as shown in the dot-and-cross diagram below
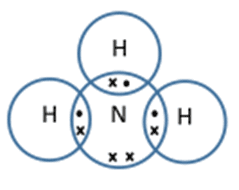
- Carbon dioxide (CO2) – carbon atoms have four electrons in their outer shell, meaning they need to gain four. Oxygen atoms need to gain two electrons. In carbon dioxide a double bond is formed between the central carbon atom and each of the two oxygen atoms. The dot-and-cross diagram for carbon dioxide is shown below
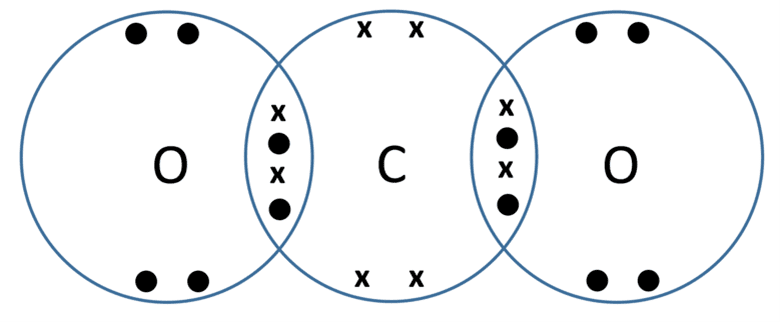
Organic molecules
- Methane (CH4) – carbon has four outer electrons. This is half a full shell, so four covalent bonds are formed to make up the outer shell. This is done with hydrogen atoms that only require one additional electron each, so there will be four hydrogen atoms and only one carbon atom, as shown in the dot-and-cross diagram below
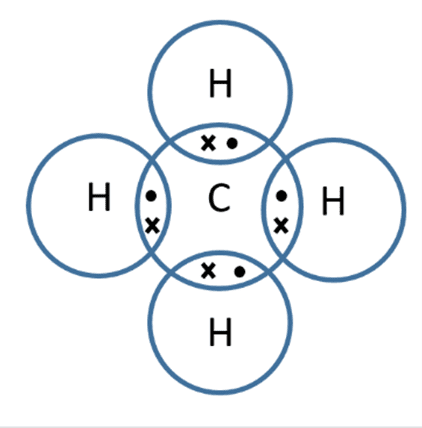
Ways of representing covalent bonds
Dot-and-cross diagrams are one way of representing covalent bonds in molecules. Another way in which they can be shown uses links between the atoms in models. These links represent a covalent bond. Below is a diagram showing how links between atoms in models is used to show covalent bonds.
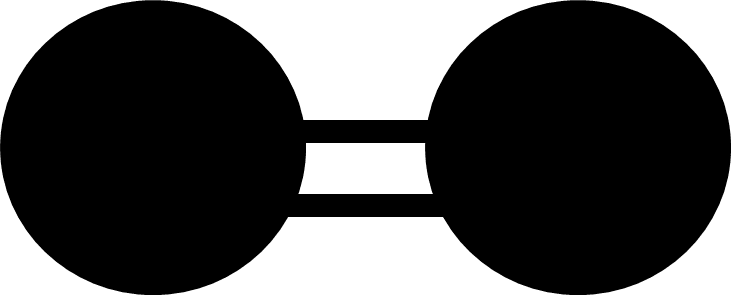
Alternatively, covalent bonds can be drawn as a straight line joining the atoms where each line refers to a pair of shared electrons. In these diagrams, sometimes you may draw the non-bonding pairs of electrons in the outer level (also known as lone pairs) and sometimes you can even leave them out. For example, the double covalent bond formed in O2 can be shown as:
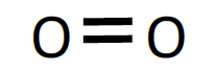
Simple molecular covalent structures
There are two types of structures formed through covalent bonding. Some covalent compounds exist as simple molecular structures whereas some exist as giant covalent structures.
Simple molecular structures
Simple molecules only contain a few atoms which are covalently bonded together. These covalent bonds inside the molecule are strong and hold the atoms together.
Forces of attraction exist between all molecules. These forces are known as intermolecular forces of attraction. As the simple molecules themselves are small, the intermolecular forces of attraction between the molecules are weak.
When a covalent bonded simple substance melts or boils, the molecules are not broken down into their atoms, they are just separated away from each other. We do not break the covalent bonds between the atoms inside the molecule.
For example, liquid water (H2O) boils to become gaseous water (H2O). The chemical formula does not change, because the covalent bonds between the oxygen and hydrogen atoms are not broken. The only change is the distance between the molecules, which increases as the forces of attraction are overcome and the molecules separate.
As the intermolecular forces of attraction are so weak in a simple covalent structure, the amount of energy required to overcome them is relatively small. This means that simple covalent structures have low melting and boiling points and are often therefore found as gases and liquids at room temperature.
The larger the simple molecular structure is, the more surface area it has and the stronger the intermolecular forces formed. The higher the strength of the intermolecular forces, the higher the amount of energy needed to overcome them and the higher the melting and boiling points will be for the substance.
As the relative molecular mass of the simple molecular compound increases, the melting and boiling points also increase.
Giant covalent structures
Giant covalent structures have a very large number of atoms arranged in a regular way and all bonded to each other by strong covalent bonds. Giant covalent structures are also known as macromolecular structures.
When melting or boiling a substance with a giant covalent structure, the covalent bonds must be broken to separate the atoms and break the structure.
As covalent bonds are so strong, and because there are so many of them in the structure, it takes a large amount of energy to break them all.
This explains why substances with giant covalent structures are usually solids at room temperature and have very high melting and boiling points.
Most giant covalent compounds do not conduct electricity as all of their electrons are used up in covalent bonds and are not free to carry charge.
Diamond, graphite and C60 fullerene are all made of carbon atoms and are all examples of giant covalent substances. However, these three substances have different physical properties due to the arrangement of their carbon atoms.
Diamond
Each carbon atom in diamond forms four covalent bonds to four other carbon atoms to form a giant structure, as shown in the diagram below.
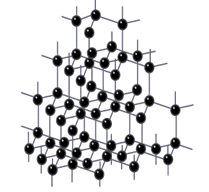
These covalent bonds are very strong and require a large amount of energy to break, and as there are so many of them theroughout the structure, diamond is a solid at room temperature with a very high melting and boiling point.
The strong covalent bonds hold the structure together firmly, so diamond is hard and very strong.
Diamond does not conduct electricity as the electrons in the outer levels of the carbon atoms are all used in covalent bonds and are not free to move around and carry electrical charge.
Diamond is insoluble in water or any other solvent as the strong covalent bonds are so strong that the solvent cannot overcome them.
Graphite
Graphite is similar to diamond in that it is a giant covalent structure made up of a large number of carbon atoms covalently bonded together. However, it differs from diamond in that is is made up of layers of carbon atoms which are able to move over each other.
As each of the carbon atoms in graphite only forms three covalent bonds to other carbon atoms, they all have a fourth electron which has not been used in bonding. This fourth electron can be delocalised from the carbon atom and is free to move and carry charge around the whole structure. This means that, unlike diamond, graphite is a good conductor of electricity and heat. The image below shows the layered structure of graphite.
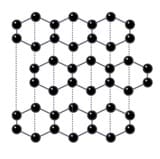
The layers of graphite are held in place by very weak intermolecular forces of attraction. As these forces are so weak, the layers are able to move over each other and can be removed. Graphite is used as the ‘lead’ in pencils. When you sharpen your pencil, you are removing layers of the graphite.
One layer of graphite is a material known as graphene. These graphene layers are very thin and are very useful for many things such as filtering other particles.
Due to the number and strength of the covalent bonds throughout the whole giant structure of graphite, it takes a lot of energy to break down the structure of the layers. Therefore, graphite has a high melting and boiling point. Graphite is also insoluble in any solvent.
In diamond, the carbon atoms are all closely packed together. In graphite, there are spaces between the carbon atoms and the layers. This means that graphite has a lower density than diamond.
C60 fullerenes
Fullerenes are giant covalent structures made up of carbon atoms covalently bonded together. C60 fullerene, also known as Buckminsterfullerene, is a specific type of fullerene which is made up of 60 carbon atoms. C60 is shaped like a football with the carbon atoms arranged in interlocking and alternate pentagons and hexagons, as shown in the diagram below:
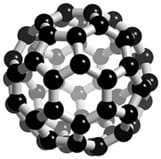
Buckminsterfullerene has a high metling and boiling point just like diamond and graphite due to the large number of strong covalent bonds between the carbon atoms in the structure. There are large gaps in the structure which means that buckminsterfullerene is not very dense and is therefore very lightweight, which makes it ideal for reinforcing structures such as tennis racquet frames where strength but lightness is a desrired characteristic. As each carbon atom in buckminsterfullerene only bonds to three other carbon atoms, they all have a fourth electron which can be delocalised. This means that buckminsterfullerene is a good conductor of electricity and heat.
Key term
Delocalised electrons – electrons which have not been used to form covalent bonds. These electrons are free to move throughout the whole structure as they are not attached to any one atom.